Glutamate
Published: Jul 17, 2023
/
Updated: Jul 30, 2023

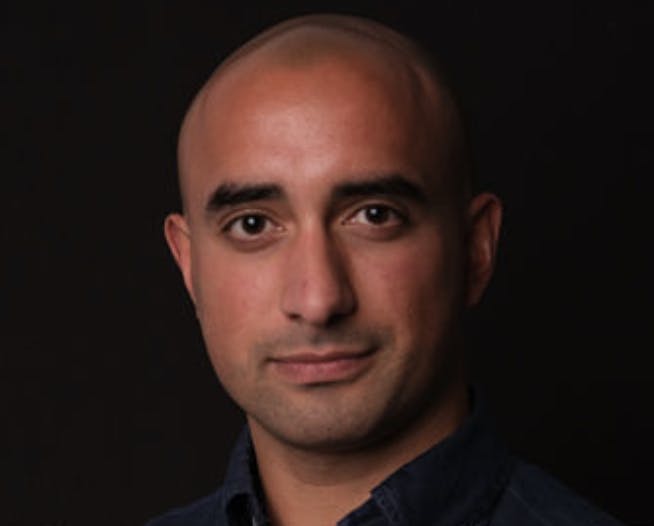
Written by Oseh Mathias
Founder, SpeechFit
Glutamate is one of the most abundant and important neurotransmitters in the central nervous system (CNS). It is an excitatory neurotransmitter, meaning it enhances or promotes the activity of neurons in the brain[1].
While gamma-aminobutyric acid (GABA) is the primary inhibitory neurotransmitter in the CNS, glutamate is the primary excitatory neurotransmitter in the CNS.

Glutamate is synthesised through various metabolic pathways. The most important of these are the Glutamine-Glutamate Cycle and the Tricarboxylic Acid (TCA) Cycle, also known as the Krebs Cycle[3].
Here's a brief overview of these processes:
Glutamine-Glutamate Cycle
In the Glutamine-Glutamate Cycle, the synthesis and recycling of glutamate occur between neurons and astrocytes. Astrocytes are a type of glial cell in the brain that play important roles in supporting neuronal function[4].
The cycle begins when glutamate is released from neurons into the synaptic cleft, the small space between neurons where neurotransmitters are released.
After glutamate has been used for signal transmission, it's taken up by astrocytes.
Within the astrocytes, an enzyme called glutamine synthetase converts glutamate into glutamine. This is a crucial step, as it detoxifies glutamate, which can be harmful to cells in high concentrations.
The glutamine is then transported back to the neuron, where it's converted back into glutamate by an enzyme called glutaminase. The glutamate is then stored in vesicles for future release.
Tricarboxylic Acid (TCA) Cycle
The TCA cycle is a series of chemical reactions used by all aerobic organisms to release stored energy[5]. This cycle also contributes to the production of glutamate in the brain.
The TCA cycle begins with a molecule called glucose, which is broken down into a molecule called pyruvate through the process of glycolysis in the cell's cytoplasm.
Pyruvate is then transported into the mitochondria, where it's converted into acetyl CoA.
Acetyl CoA enters the TCA cycle and goes through a series of reactions. One of the intermediates of the TCA cycle is a molecule called alpha-ketoglutarate.
Alpha-ketoglutarate is transaminated, meaning it receives an amino group (-NH2) to become glutamate. This reaction is catalyzed by an enzyme called aspartate aminotransferase (AST) or alanine aminotransferase (ALT), which transfer the amino group from aspartate or alanine to alpha-ketoglutarate.
These pathways allow for the constant synthesis and recycling of glutamate in the brain, maintaining an adequate balance for proper neural function.
Glutamate can also be used to produce gamma-aminobutyric acid (GABA), the main inhibitory neurotransmitter[6].
A very simplified version of the process of conversion of glutamate to GABA occurs through the following steps:
Decarboxylation of Glutamate: The enzyme glutamate decarboxylase (GAD) catalyzes the decarboxylation of glutamate to GABA. This reaction requires the presence of the coenzyme pyridoxal phosphate (PLP), which is the active form of vitamin B6.
Conversion to GABA: The reaction with glutamate decarboxylase removes a carboxyl group from glutamate, converting it into GABA.
You can read more about GABA synthesis here.
Glutamate is involved in a wide range of brain functions, including learning, memory, cognition, and the regulation of neural plasticity (the brain's ability to change and adapt). It plays a crucial role in synaptic transmission, which is the process by which neurons communicate with each other.
Once synthesised, glutamate is stored in vesicles within the presynaptic neuron and released into the synapse upon stimulation. It then binds to and activates glutamate receptors located on the postsynaptic neuron, initiating a cascade of biochemical reactions that lead to the transmission of signals between neurons.

Glutamate receptors are categorised into two main types: ionotropic receptors and metabotropic receptors[8].
Ionotropic receptors, such as NMDA, AMPA, and kainate receptors, are ligand-gated ion channels that directly allow ions to flow into the postsynaptic neuron when glutamate binds to them. This rapid influx of ions triggers electrical signals in the postsynaptic neuron, leading to excitatory effects.
Metabotropic receptors, on the other hand, are coupled to intracellular signaling pathways through G proteins. Activation of metabotropic glutamate receptors modulates neuronal activity indirectly, influencing various cellular processes and neuronal excitability.

While glutamate is essential for normal brain function, excessive glutamate release or impaired regulation of glutamate signalilng can lead to excitotoxicity—a state of neuronal damage and cell death caused by overstimulation of glutamate receptors[10]. Excitotoxicity has been implicated in several neurodegenerative disorders and neurological conditions, such as stroke, Alzheimer's disease, and Parkinson's disease.
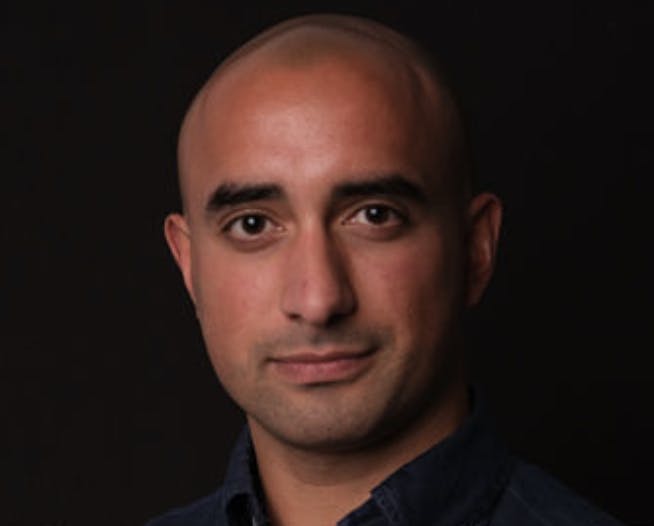
Oseh is a software engineer, entrepreneur and founder of SpeechFit. Oseh is passionate about improving health and wellbeing outcomes for neurodiverse people and healthcare providers alike.
References
Meldrum, B. S. (2000). Glutamate as a neurotransmitter in the brain: Review of physiology and pathology. Journal of Nutrition, 130(4S Suppl), 1007S–15S.
Bak, L. K., Schousboe, A., & Waagepetersen, H. S. (2006). The glutamate/GABA-glutamine cycle: Aspects of transport, neurotransmitter homeostasis and ammonia transfer. Journal of Neurochemistry, 98(3), 641–653.
Sofroniew, M. V., & Vinters, H. V. (2010). Astrocytes: biology and pathology. Acta Neuropathologica, 119(1), 7–35.
Alberts, B., Johnson, A., Lewis, J., Raff, M., Roberts, K., & Walter, P. (2002). Molecular Biology of the Cell. New York: Garland Science.
Petroff, O. A. C. (2002). GABA and Glutamate in the Human Brain. The Neuroscientist, 8(6), 562–573.
Martin, D. L., & Rimvall, K. (1993). Regulation of Gamma-Aminobutyric Acid Synthesis in the Brain. Journal of Neurochemistry, 60(2), 395–407.
Satarker, S., Bojja, S. L., Gurram, P. C., Mudgal, J., Arora, D., & Nampoothiri, M. (2022). Astrocytic Glutamatergic Transmission and Its Implications in Neurodegenerative Disorders. Cells, 11(7), 1139. Figure 1. Glutamate homeostasis at the tripartite glutaminergic synapse. Retrieved from https://doi.org/10.3390/cells11071139
Traynelis, S. F., Wollmuth, L. P., McBain, C. J., Menniti, F. S., Vance, K. M., Ogden, K. K., ... & Dingledine, R. (201
Salt, T. (n.d.). Properties of glutamate receptors. In Neurotransmitters in Sensory Systems. University College London. Retrieved July 26, 2023, from https://www.ucl.ac.uk/~smgxt01/frameh.htm?page=glutamat.htm
Choi, D. W. (1988). Glutamate neurotoxicity and diseases of the nervous system. Neuron, 1(8), 623-634.