Mesocortical Pathway
Published: Jul 18, 2023
 / Â
Updated: Jul 29, 2023
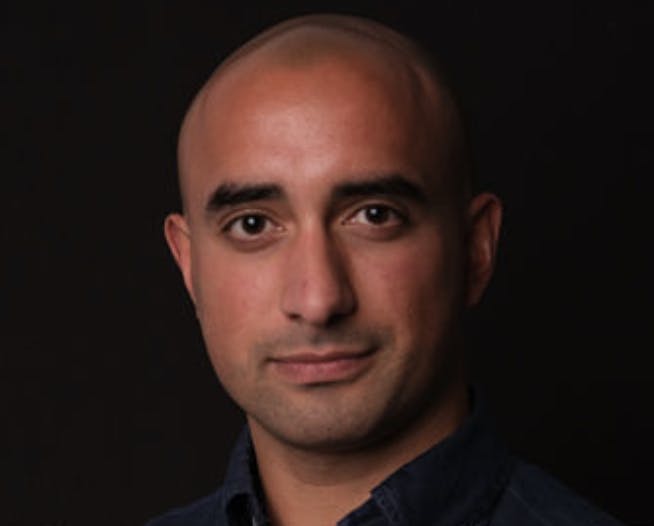
Written by Oseh Mathias
Founder, SpeechFit
The mesocortical pathway is one of the major dopamine pathways in the brain[1]. The term "mesocortical" refers to the source and destination of this pathway – "meso" stands for "mesencephalic" (the technical term for the midbrain), and "cortical" refers to the cerebral cortex.
The mesocortical pathway originates in the ventral tegmental area (VTA) of the midbrain. From the VTA, dopaminergic neurons project and release dopamine into various regions of the cerebral cortex[2].
The primary function of the mesocortical pathway is associated with cognition and emotion, with a particular focus on motivation and emotional response [4]. This pathway plays an essential role in the so-called "reward system" of the brain. It is involved in the regulation of executive functions, such as working memory, decision making, and attention [5].
The mesocortical pathway primarily interacts with the prefrontal cortex, a region critical for higher cognitive functions [6].
How does the mesocortical pathway influence cognition?
Neurons in the VTA produce dopamine. These neurons send projections via the mesocortical pathway to the prefrontal cortex, releasing dopamine there [7]. Dopamine release in the prefrontal cortex influences cognition by playing a role in working memory and attention - if you recall the definition of cognition, it is highly dependent on working memory to process information [8].
Working memory, which is responsible for temporarily holding and processing information, relies on the sustained and coordinated activity of groups of neurons in the prefrontal cortex (PFC) [9].
Dopamine's influence on working memory
Signal-to-Noise Ratio: A key concept in neural processing is the "signal-to-noise ratio", which is essentially the differentiation between relevant (signal) and irrelevant (noise) information [10]. The prefrontal cortex is a highly associative area of the brain, receiving inputs from various sensory modalities and other cortical areas. These various inputs can be thought of as "signals" that need to be distinguished from "noise." Dopamine is thought to modulate the responsivity of PFC neurons in a way that amplifies the "signal" (i.e., relevant information) while suppressing the "noise" (i.e., irrelevant information). This can be crucial for maintaining the integrity of information in working memory [11].
The key is that dopamine does not have a uniform effect on all neurons or even on all parts of a single neuron. The impact of dopamine depends on various factors, including the type of dopamine receptor the neuron expresses, the location of these receptors on the neuron, and the current state of the neuron (i.e., whether it is firing action potentials or not) [12].
Dopamine works on the signal-to-noise ratio in the following ways:
Signal Amplification: Dopamine has been found to enhance the 'signal' by increasing the responsivity of certain neurons in the PFC to inputs that they are already responding to [13]. Dopamine can make these neurons more likely to fire in response to these inputs, essentially making the 'signal' stronger. This can help maintain the activity of neural circuits that are holding relevant information in working memory [14].
Noise Suppression: At the same time, dopamine can decrease the responsivity of these neurons to other, 'noisy' inputs that they are not already responding to. This makes it less likely that these neurons will be distracted by irrelevant information, effectively reducing the 'noise' [15].
Molecular breakdown of dopamine's effect on cognition via the mesocortical pathway
The prefrontal cortex contains two main types of dopamine receptors: D1 and D2. These receptors have different effects on neurons[16].
D1 receptors: These are generally excitatory, meaning that when they are activated by dopamine, they can increase the neuron's firing rate. They are often found on the 'body' (soma) and the main output branches (axon) of neurons. By activating D1 receptors, dopamine can increase the 'signal' by enhancing the firing of neurons in response to inputs they are already responding to[17].
D2 receptors: These are generally inhibitory, meaning that when they are activated by dopamine, they can decrease the neuron's firing rate. They are often found on the input branches (dendrites) and the synaptic terminals of neurons. Activation of D2 receptors can help suppress the 'noise' by reducing the likelihood that the neuron will respond to 'noisy' inputs it receives[18].
Modulation of other neurotransmitters: Dopamine can also influence the action of other neurotransmitters. For example, it can modulate the function of GABA (an inhibitory neurotransmitter) and glutamate (an excitatory neurotransmitter) neurons, thereby indirectly affecting the generation of action potentials[18].
By selectively enhancing the firing of some neurons (those holding relevant information) and inhibiting the firing of others (those processing irrelevant 'noise'), dopamine can help to maintain the integrity of information in working memory, thus improving cognitive performance[19].
The net effect of dopamine on a particular neuron in the PFC will depend on the balance of these different actions, as well as other factors like the current state of the neuron and the presence of other neurotransmitters[20].
Other effects of the mesocortical pathway on cognition
Neuronal Excitability and Connectivity: Dopamine also affects the excitability of neurons, i.e., how likely they are to fire. This modulatory effect can influence the connectivity between different neurons involved in a working memory task. Dopamine release can make certain neural pathways more likely to be used, thereby affecting the way information is processed and stored[21].
Long-Term Potentiation (LTP): Dopamine is involved in the process of Long-Term Potentiation, which is a long-lasting enhancement in signal transmission between two neurons after synchronous stimulation. This mechanism is thought to be a key process in learning and memory. Dopamine can enhance LTP, thereby strengthening the connections between neurons and helping to consolidate the neural patterns that correspond to the information being held in working memory[22].
The mesocortical pathway is one of the four major dopamine pathways in the brain, the others being the mesolimbic pathway, the nigrostriatal pathway, and the tuberoinfundibular pathway[23].
The mesocortical and the mesolimbic pathways are often mentioned together because they both originate from the VTA. While the mesocortical pathway projects to the cerebral cortex, the mesolimbic pathway projects to the limbic system. These two pathways are central to the brain's reward circuit and are also implicated in several psychiatric conditions, including schizophrenia, mood disorders, and substance use disorder[24].
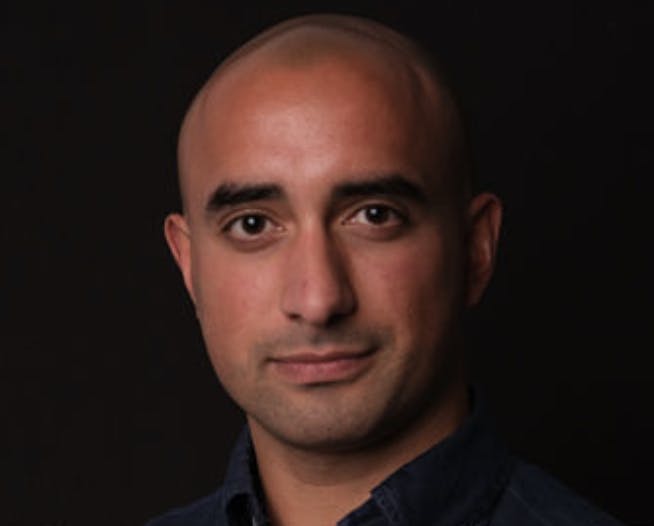
Oseh is a software engineer, entrepreneur and founder of SpeechFit. Oseh is passionate about improving health and wellbeing outcomes for neurodiverse people and healthcare providers alike.
References
Wise, R. A. (2008). Dopamine and reward: The anhedonia hypothesis 30 years on. Neurotoxicity Research, 14(2-3), 169-183.
Bäckman, L., Nyberg, L., Lindenberger, U., Li, S. C., & Farde, L. (2006). The correlative triad among aging, dopamine, and cognition: Current status and future prospects. Neuroscience & Biobehavioral Reviews, 30(6), 791-807.
Slashme, Patrick J. Lynch, & Fvasconcellos. (2015). The main dopaminergic pathways of the human brain: the mesocortical pathway, connecting the ventral tegmental area (VTA) with the frontal cortex; the mesolimbic pathway, connecting the VTA with the nucleus accumbens; the nigrostriatal pathway, connecting the substantia nigra with the dorsal striatum; and the tuberoinfundibular pathway, connecting the hypothalamus with the pituitary [Image]. Wikimedia Commons. https://commons.wikimedia.org/wiki/File:Dopaminergic_pathways.svg
Durstewitz, D., & Seamans, J. K. (2008). The dual-state theory of prefrontal cortex dopamine function with relevance to catechol-o-methyltransferase genotypes and schizophrenia. Biological psychiatry, 64(9), 739-749.
Fuster, J. M. (2001). The prefrontal cortex—an update: time is of the essence. Neuron, 30(2), 319-333.
Grace, A. A. (2016). Dysregulation of the dopamine system in the pathophysiology of schizophrenia and depression. Nature Reviews Neuroscience, 17(8), 524.
Seamans, J. K., & Yang, C. R. (2004). The principal features and mechanisms of dopamine modulation in the prefrontal cortex. Progress in neurobiology, 74(1), 1-57.
Floresco, S. B. (2013). Prefrontal dopamine and behavioral flexibility: shifting from an"inverted-U" toward a family of functions. Frontiers in neuroscience, 7, 62.
Cools, R., & D'Esposito, M. (2011). Inverted-U–shaped dopamine actions on human working memory and cognitive control. Biological psychiatry, 69(12), e113-e125.
Lisman, J. E., & Grace, A. A. (2005). The hippocampal-VTA loop: controlling the entry of information into long-term memory. Neuron, 46(5), 703-713.
Arnsten, A. F. T. (2009). Stress signalling pathways that impair prefrontal cortex structure and function. Nature Reviews Neuroscience, 10(6), 410-422.
D'Esposito, M., & Postle, B. R. (2015). The cognitive neuroscience of working memory. Annual review of psychology, 66, 115-142.
Baddeley, A. (2003). Working memory: looking back and looking forward. Nature reviews neuroscience, 4(10), 829-839.
Gold, J. M., & Weinberger, D. R. (1995). Cognitive deficits and the neurobiology of schizophrenia. Current opinion in neurobiology, 5(2), 225-230.
Volkow, N. D., & Morales, M. (2015). The brain on drugs: from reward to addiction. Cell, 162(4), 712-725.
Beaulieu, J. M., & Gainetdinov, R. R. (2011). The physiology, signaling, and pharmacology of dopamine receptors. Pharmacological Reviews, 63(1), 182–217.
Frank, M. J., & O'Reilly, R. C. (2006). A mechanistic account of striatal dopamine function in human cognition: Psychopharmacological studies with cabergoline and haloperidol. Behavioral Neuroscience, 120(3), 497–517.
Tritsch, N. X., & Sabatini, B. L. (2012). Dopaminergic modulation of synaptic transmission in cortex and striatum. Neuron, 76(1), 33–50.
Durstewitz, D., & Seamans, J. K. (2008). The dual-state theory of prefrontal cortex dopamine function with relevance to catechol-o-methyltransferase genotypes and schizophrenia. Biological Psychiatry, 64(9), 739–749.
Seamans, J. K., & Yang, C. R. (2004). The principal features and mechanisms of dopamine modulation in the prefrontal cortex. Progress in neurobiology, 74(1), 1-57.
Gulledge, A. T., & Jaffe, D. B. (2001). Dopamine decreases the excitability of layer V pyramidal cells in the rat prefrontal cortex. Journal of Neuroscience, 21(21), 8655–8665.
Lisman, J., & Grace, A. A. (2005). The hippocampal-VTA loop: controlling the entry of information into long-term memory. Neuron, 46(5), 703–713.
Haber, S. N. (2014). The place of dopamine in the cortico-basal ganglia circuit. Neuroscience, 282, 248-257.
Nestler, E. J., & Carlezon Jr, W. A. (2006). The mesolimbic dopamine reward circuit in depression. Biological Psychiatry, 59(12), 1151–1159.